Ultra-Cold Neutron Source

Ultra Cold Neutrons
Ultracold neutrons (UCN) are neutrons moving so slowly that their kinetic energies is comparable to their potential energies in earth’s gravitational field. Such low energies, or equivalently long wavelength (10’s of nm), lead to quite unusual behavior compared to cold, thermal or fast neutrons. The most dramatic difference is that, unlike more common higher energy neutrons that penetrate easily through solids or liquids, UCN can be totally reflected at any angle of incidence from surfaces of almost any material. They behave like a gas, filling the available volume in material or magnetic traps:

The traps shown above give an idea of the gravitational (2 foot rise) versus magnetic or material trapping fields. If the kinetic energies of the neutrons exceeds the trapping field even slightly they can escape, becoming “normal” penetrating neutrons. Nevertheless, they can enter the walls only within surface nanolayers, because the interaction cross section is inversely proportional to the neutron velocity ( “1/v” law ) and becomes enormous for the UCN energy range.
The trapping of neutrons is extremely interesting for fundamental particle physics – you can get answers to some fundamental questions about our universe. For example: “What is the reason for the matter/antimatter asymmetry?” In these experiments, the longer observation time and low velocities of the neutrons leads to a significant increase of experimental sensitivity.
For material applications, the strong selective interaction within the surface layer makes the UCN a unique tool to probe surface elemental composition. This can be used as an extension of prompt gamma activation analysis, PGGA, and depth profiling. For many absorbing materials even submonolayer traces can be detected! In addition, UCN can probe the molecular dynamics of the hydrogenated surface layers, which is of interest for molecular membrane and nanocoating research. These will be exciting projects for our facility in the near future.
To convert neutrons produced in the reactor into UCN, one has to decelerate them to almost zero speed, say, 10 feet per second – similar to that of a running person. Now, the average energy of fission neutrons is about 10+6 eV – compared with the UCN energy of about 10-7 eV. So this is what our facility is doing – reducing the neutrons’ energies by 13 orders of magnitude.
How are we doing it? Similar to how one cools a boiled egg – by placing hot neutrons into something cold and big enough to hold them until their temperature equilibrates with the media. This process is called “neutron moderation”. Of course, it is a little more complicated because of such a huge range of deceleration. Neutronic modeling shows that the most optimal design is the 3-step moderation process shown below:

First, hot neutrons from fission are cooled by heavy water to room temperature, after which they are further cooled by entering solid methane at around 45K and become “cold neutrons”. In these two steps, neutrons are cooled by many collisions, each one taking away some energy, similar to how water molecules take away energy from the cooled egg. But at the end they will enter solid deuterium, where the analogy with an egg is no longer valid. Here some of neutrons will loose practically all of their kinetic energy (five orders of magnitude!) at once. This is a type of resonance effect, which happens only when the kinetic energy of the neutron matches one of the possible excitation energies of the deuterium crystal. When this happens, the neutron excites otherwise frozen motion of the molecules and as result, loses all its energy and becomes “ultracold”, with effective temperature of about 1 mK compared to the solid deuterium temperature of 5K. Since ultracold neutrons are not in thermal equilibrium with deuterium, they can be easily heated back up by their next collision. To prevent this, they have to be extracted as quickly as possible from the deuterium crystal. This means that the UCN producing material should be rather thin and placed inside a UCN guide to deliver the neutrons to an experiment.
After learning a little about the principles of UCN source operation, let’s see how it looks in reality. The picture below shows a conceptual neutronic geometry of our facility, designed by Dr. B. Wehring (more details see in publications below).

The reactor core is where the hot fission neutrons are produced. Graphite serves as a reflecting channel to deliver them to the moderators, which are placed outside the reactor pool. The heavy water (D2O) moderator fills a large tank, in which an elbow of the UCN guide is immersed. At the very bottom, the UCN guide contains the deuterium crystal and is surrounded by a cap-shaped methane container. Since both the deuterium and the methane have to be cooled down to low temperatures, this part of the facility has to be designed as a cryostat, i.e. a device which allows its inner parts to be at significantly lower temperatures than an outside shell. The next picture shows a section of the 3D engineering design of our source cryostat:

Both methane and deuterium are cooled by flows of liquid helium through the corresponding heat exchangers. The neutron guide above the deuterium container has to be held at a temperature above 50K to prevent deuterium condensation on its walls (deuterium condenses around 18K). To thermally disconnect the two parts of the cryostat a zircalloy section, which has very low thermal conductivity, is right above the deuterium. 3D mesh structures made of the titanium alloy Ti6Al4V, which have an even lower thermal conductivity, will be used as spacers to fix the position of the inner cold elbow inside the warm vacuum shield. Notice that the choice of materials takes into account their resistivity to neutron and gamma radiation (i.e. no plastics) and neutron activation properties, because we would like to have the possibility of working with and improving the cryostat after it is commissioned inside the reactor and exposed to the neutron flux.
The total neutron flux inside deuterium is expected to be about 5×1011 n/sec/cm2. From this amount we expect to convert 104 of them per second into the UCN energy range, and 30% of them should make it to the position of experiments, which is indeed quite efficient: our 3-step moderation will provide a useful density at least 30 UCN/cc per 1MW of reactor power as compared with about 1 UCN/cc per 1 MW at the existing UCN facility at ILL, France.
Finally, you can see a real cryostat (without the outer shell) inside the heavy water tank:

Project Schedule and Milestones
Project Schedule:
- Optimization of the solid ortho-deuterium growth – Spring 2017.
- Installation of the temporarily shield – May 2017.
- Assembling for permanent installation – June 2017- June 2018.
- Radiation shield testing – Summer 2018.
- UCN production test – Fall 2018.
Project Milestones:
- Chill water loop modification, step 1 – completed Feb. 2017.
- Manufacturing temporary shield required during final assembling of the cryostat on the biological shield door – Completed December 2016.
- Cryogenic commissioning of the deuterium system with deuterium to study solid ortho-deuterium growth – First run was completed in Spring 2016.
- Cryogenic commissioning of the deuterium system; first with neon and then with para-deuterium – Completed in 2015.
- Cryogenic commissioning of the methane system (using LHe for cooling the cryostat); first with nitrogen and then with methane, and optimization of procedure for solid methane growth – Completed in 2015.
- Cryostat Test assembling: complete assembly of the neutron guide vacuum jacket with the cryostat in place on the supporting frame – performed successfully in June 2013.
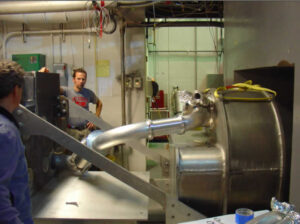
Thermal Column Insertion Test – June 2013
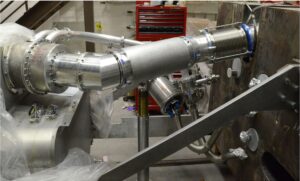
Neutron Guide Assembly – June 2013
- External safety review for overall design and methane and deuterium handling procedures – Completed in January 2013.
- Cold leak testing with LN2 in place of LHe and test condensation of argon in place of methane – Completed in 2012.
- Manufacturing of the cryostat parts and assembling of the cryostat in preparation for the first cold leak test – Completed in December 2011.
- Manufacturing and assembling of the gas handling system – Completed in December 2011.
- Commissioning of the D2O system and MCNP benchmark measurements using a test water tank filled first with H2O (Phase I) and then with D2O (Phase II). The test tank was designed to measure the neutron flux spacial distribution using gold foils. This step was required for estimation of the radiation background outside the bioshield and for better understanding of the physics of UCN production – Completed in July 2010.
- Installation and Commissioning of the beam line components including measurement of the experiment reactivity value with shielding box and nose port in place – Completed in 2010.
- Modification of the former thermal column cavity, including installation of the supporting frame on the thermal column door and verifying cryostat position relative to the door – Completed in Spring 2010.
- Commissioning of the LHe liquefier – Completed in 2008.
Please contact the Manager of Nuclear Services if you are interested in learning more about the Ultra Cold Neutron Source.
Related Publications
Conference presentations:
- PSI2016, speaker E. Korobkina, ”PULSTAR UCN Source: Studying solid deuterium growth and evolution”; Nov 2016, Paul-Scherrer-Institute, Switzerland.
- APS DNP2016, speaker Christian White, “Cryogenic testing of the PULSTAR UCN Source” , October 2016, Vancouver, USA
- International Workshop on Probing Fundamental Symmetries with UCN; 11-15 April 2016, JGU Mainz, Germany; speaker E. Korobkina, “Design and results of cryogenic commissioning tests of the PULSTAR UCN source”.
- International Workshop on “Challenges of the world-wide experimental search for the electric dipole moment of the neutron”, Nov 2014, Ascona, Switzerland , speaker E. Korobkina, “PULSTAR UCN source and EDM Systematic Study”.
Publications:
- Korobkina,G. Medlin, B. Wehring, A.I. Hawaii, P.R. Huffman, A.R. Young, B. Beaumont, G. Palmquist; “ Ultracold neutron source at the PULSTAR reactor: Engineering design and cryogenic testing”; Nuclear Instruments and Methods A, vol. 767, 2014, 169-175
- Korobkina, B. Wehring, A. Hawari, A. Young, P. Huffman, R. Golub, Y. Xu, G. Palmquist; “An Ultracold Neutron Source at the NC State University PULSTAR Reactor”; Nuclear Instruments and Methods A, 2006.
White papers:
- Golub, H. Gao, D. Haase, P. Huffman, A. Merizalde, C. Swank, Q. Ye, TUNL; A. Hawari, E. Korobkina, NC State University Nuclear Engineering Department, Raleigh, NC; S. Lamoreaux, Yale University; “Systematic Studies for the nEDM Experiment at the PULSTAR UCN Facility”; The NEDM Collaboration
Posters:
- 2014 Symposium on Radiation Measurements and Applications (SORMA XV), Ann Arbor, Michigan USA, presenter Graham Medlin, “Testing the Ultracold Neutron Source at the PULSTAR Reactor”
- “The NC State University PULSTAR Reactor UCN Source” ; Jan. 16th, 2012