Neutron Powder Diffraction Facility
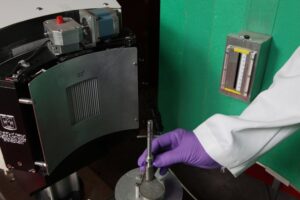
Neutron Diffraction – A Tool for Materials Science
In the development of new materials, the goal is usually to understand the connection or correlation between material properties and materials structure. The stepping stones to this understanding are the fundamental crystal structure, the position and chemical species of the constituent atoms, the species, location, concentration of impurities and the nature of the specimen structural defects.
How it Works
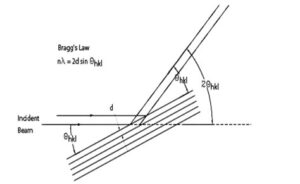
When a polycrystalline specimen is bathed in a monochromatic (single wavelength) beam of neutrons, there are always many grains of the sample that are aligned to reflect the radiation according to Bragg’s Law – See Fig #1. The diffracted neutrons form cones of radiation corresponding to the many different atom plane spacings (d) in the crystals – See Fig #2.
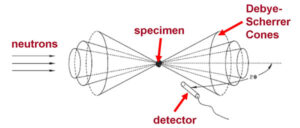
If a detector is arranged to scan through the scattered radiation, a series of peaks appears as a function of angle. The positions of the peaks (in angle) is determined, through Bragg’s Law, by the spacing of atoms in the specimen crystal structure. The intensity of the diffraction peaks is controlled by the positions and atomic species of the atoms in the unit cell (the fundamental crystal building block).
Using a technique called Rietveld Refinement, the diffraction pattern calculated from the model of the crystal structure compared to the data and the atomic positions, species and thermal vibrations in the model are adjusted until a good agreement with the experimental data is obtained. The result is the “refined” crystal structure for the specimen – Example – See Figures #3 and #4. Here the structure of ettringite, formed during the setting of ordinary Portland cement has been determined.
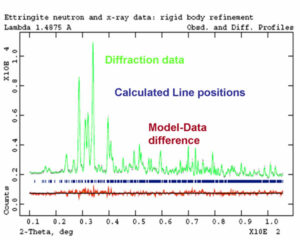
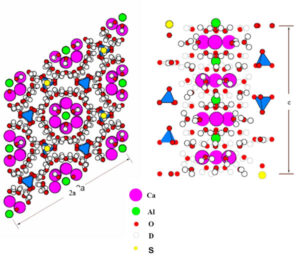
Neutrons are Wonderful !
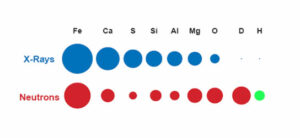
Although superficially similar to x-Ray diffraction, neutron diffraction has many advantages:
- Contrast. The neutron scattering strength is not dependent on the atomic number. It is possible to observe the effect of light elements in the presence in heavy ones in the diffraction pattern.
- Isotopes. With neutrons the strength of scattering is different for different isotopes so that isotopic substitution can be used to clairy elements of a crystal structure
- Special Environments. Neutrons are deeply penetrating so they can diffract off specimens contained in cryo-refrigerators or furnaces, making it easy to examine materials under special conditions and in special environments.
- Calculable. Unlike the case for x-Rays, the scattering of neutrons from materials can be accurately calculated making comparison to theoretical.
- Magnetism. Neutrons have a magnetic moment and their scattering is sensitive to the magnetic moment of atoms making neutron scattering the ideal means to study magnetic materials.
Characteristics of NCSU Neutron Powder Diffractometer Facility:
- Variable resolution (dd/d = 1×10-3 or 2×10-3)
- Si bent perfect crystal focusing monochromator 1.478 A
- 90° take off angle, 5° – 125° scattering range
- Detector to specimen distance: 1.6m for high resolution, 1.15m for high speed.
- Data acquisition 8 hours/pattern (high resolution), 2 hours/pattern (high speed).
- Large area position sensitive neutron detector array 15″ x 24″ spanning 20° for high resolution or 30° for high speed.
- Air-pad supported detector base.
Instrument Layout
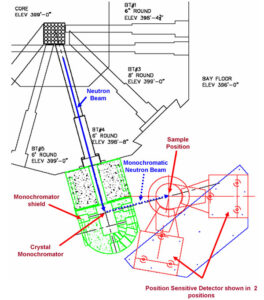
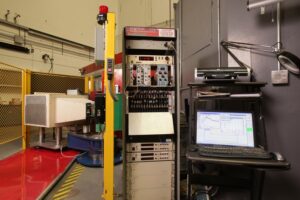
Bent Perfect Crystal Monochromator
Focusing in the vertical plane is like a simple concave mirror. Focusing in the horizontal plane is by diffraction – i.e. the beam from the sample is focused on the detector.
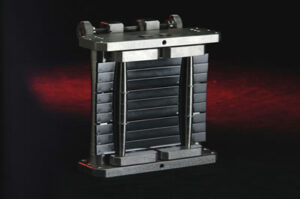
- 9 blades made from single crystal silicon plates 5.7 mm thick.
- Plates cut obliquely with <110> zone axis vertical.
- Vertical radius of curvature 1.15m
- Horizontal radius of curvature 12.25m
Neutron Detector Array
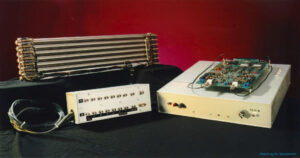
The PNPD detector contains 15 neutron linear position sensitive proportional counters (similar to the 7-element array shown here). The detectors have an active length of 24″, a diameter of 1″ and are filled with 8 Atm 3Helium and 4 Atm Argon. Neutron detection is through the reaction: n + 3He –> 1H + 3H + 768 keV
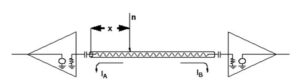
The event position utilizes the charge division method. The neutron capture event injects charge on the detector anode which divides via the anode resistance resulting in signals from the preamplifiers connected to each end of the detector element. A simple model is sufficient to show the quotient of the preamplifiers signals yields the event position. The signals from the two preamplifiers connected to each detector element are processed by a custom microprocessor controlled dual-amplifier / dual-ADC circuit board that calculates and histograms the event positions and transfers these to the host computer on command.
Diffractometer Applications
Neutron diffraction data can be directly compared to theoretical models of crystal structure and composition. This opens a wide variety of possible applications:
- New material crystal structure and composition – In the development of new materials, the correlation of structure, synthesis conditions and properties is essential.
- Alloy atom location – The intensity of diffraction peaks is directly related to the atom species and fractional occupation of the crystal atom.
- Solid – Solid phase transformation – Many materials change their crystal structure in response to changes in their environment (temperature and pressure). New crystal phases result in new diffraction peaks – the unmistakable signature of phase transformation.
- Non-contact temperature measurement – The height of diffraction peaks is modulated by atomic thermal vibrations. Once calibrated, this can be an accurate means to measure temperatures when no other method is available.
- Chemical and solid-state reaction pathways and kinetics – Many chemical and solid-state reactions can be monitored quantitatively through the intensity of the diffraction peaks corresponding to the various components.
- Material stress – strain analysis – When crystalline materials are put under stress, the spacing between their atomic planes is changed. This results in a shift of the diffraction line positions. With neutron diffraction it is possible to probe material stress distributions in real engineering materials.
- Multiphase composition analysis – Many complex materials are mixtures – not alloys. Neutron diffraction measurements combined with Rietveld refinement can be used to analyze the composition and structure of the individual components.
Intensity Comparison
Neutron flux measurement at the NPDF sample position provide a very favorable comparison to existing instruments at other neutron beam laboratories:
|
We welcome requests for measurements and collaborations from both academic institutions and industrial partners. Please contact the Manager of Nuclear Services if you are interested in learning more about applications of the neutron powder diffractometer.
Related Publications
- D. DuJulio, A. Hawari; “Examination of reactor grade graphite using neutron powder diffraction.”; Journal of Nuclear Materials, 2009.